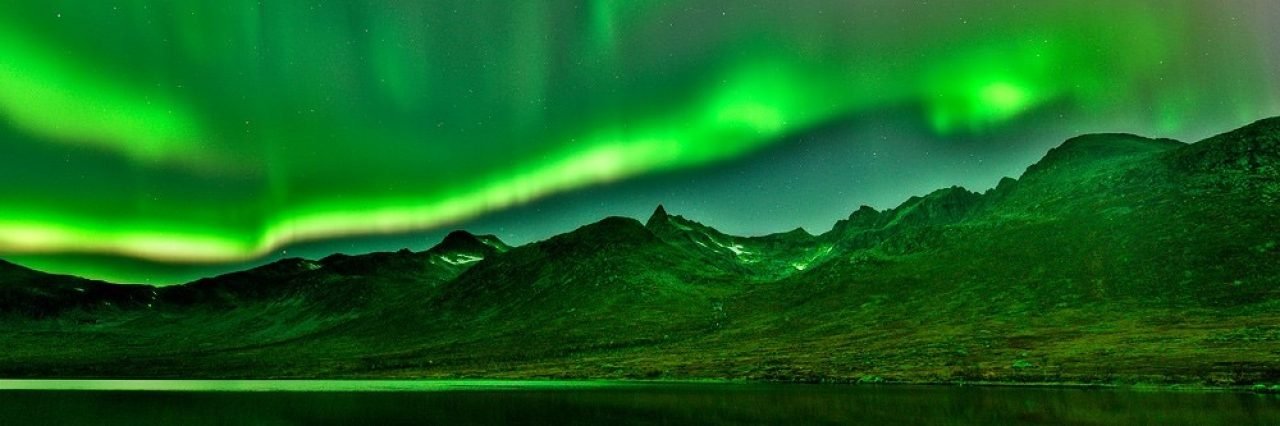
News
November 25, 2024
Space Waves Team Up to Heat Solar Wind Particles
written by Emmanuel Masongsong
UCLA Dept. of Earth, Planetary, and Space Sciences
Artist depiction of the solar wind (yellow, left) interacting with Earth's magnetosphere (blue right). At this interface, large plasma waves can form (inset at left), generating ion beams (yellow arrows) that in turn cause plasma heating to form ion acoustic waves (red). Credit: X. An, E. Masongsong, UCLA EPSS
This finding solves a long-standing puzzle about how energy moves between different scales in space plasmas and how particles are heated in these environments. It's similar to how ocean waves can create smaller ripples that eventually break and heat the water. The discovery helps explain how streaming particles from the Sun- the solar wind- are heated as they travel through space. This process of energy transfer can occur at other astrophysical settings, from the Sun's atmosphere to fusion devices on Earth. This improved understanding could help scientists better predict space weather and advance the development of fusion energy.
The new study uses detailed spacecraft observations to reveal that a large wave’s motion can speed up another smaller, separate type of wave, analogous to laboratory plasma experiments on Earth. However the scale of these wave-heating effects in lab experiments is far too small and rapid to study with terrestrial technology. In contrast, the enormous size of plasma waves in the Earth’s magnetic field magnifies this same effect such that it can be directly observed by dedicated satellites, making it possible to see these processes in real time as reported in the study.
“Fleets of spacecraft are needed to sample the fabric of space itself: plasmas that make up our sun, and the vast majority of the universe,” said lead author Xin An, a researcher in the Earth, Planetary, and Space Sciences department. It is important to investigate the nature of these particles, since plasma wave heating can impact our satellite technology and astronauts, who are especially more vulnerable on the moon, outside of our planet’s protective magnetic bubble.
X. An, et al., “Cross-scale energy transfer from fluid-scale Alfvén waves to kinetic-scale ion acoustic waves in the Earth’s magnetopause boundary layer,” Phys. Rev. Lett. (2024), 133, 225201, doi: https://doi.org/10.1103/PhysRevLett.133.225201. Published 25 November, 2024
The improved understanding of how waves work in space could help scientists better predict space weather and advance the development of fusion energy.
Waves are a common means of transferring energy from one area or system to another. In space, waves occur in plasmas - very sparse collection of atomic particles – where vibrations can travel through the plasmas’ magnetic fields. Now, Scientists have discovered a new way for different types of waves in space to work together, to heat and accelerate particles in the solar wind.
Using NASA's Magnetospheric Multiscale spacecraft and computer simulations, UCLA researchers found that large-scale magnetic waves (~2000km), known as Alfvén waves, can generate smaller-scale sound-like waves (50-1500m) that heat particles to high temperatures. This process occurs near boundaries in space where different plasma environments meet, such as at Earth's magnetopause - the outer edge of our planet's magnetic shield. The research is published in the latest issue of Physical Review Letters.
June 6, 2024
ELFIN Student Team wins NASA TechLeap Prize
ELFIN student team
UCLA’s ELFIN student team, part of the Experimental Space Physics (ESP) lab of Professor Vassilis Angelopoulos, is one of the three finalists winning the 2024 NASA TechLeap Prize, a challenge to develop interface systems that easily integrate diverse space payloads onto flight vehicles.
The Experimental Space Physics lab focuses on in situ measurements of plasma, particles, and fields in near-Earth space. The sizable undergraduate student portion of the lab is led by Project Manager Ethan Tsai, who previously oversaw the successful completion of the NASA ELFIN CubeSat mission (elfin.epss.ucla.edu). Sophie Ye, a graduating senior, will lead the focused undergraduate team's development of the UCLA Software-Defined Payload Interface (SDPI), which leverages previous flight experience and syngerizes well with future EPSS ambitions for spaceflight.
Mentored by graduate students and engineering staff at EPSS, the SDPI team aims to reduce the cost and complexity of integrating scientific payloads into orbital vehicles and lunar landers which solves a key bottleneck in the performing scientific investigations in space. Their proposal for the Software-Defined Payload Interface (SDPI) is powered by a flight-proven ARM/FPGA System-on-Chip and provides customers with fleixbile user interface that allows for the configuration of communication and power interfaces for seamless integration into a wide range of space applications. The SDPI team will have access to a fully-equipped electrical engineering lab, the EPSS prototyping shop, and a fully-operational, in-house thermal-vacuum chamber (TVAC), and will work alongside other like-minded students building space plasma instrumentation and satellite buses.
May 30, 2024
Professor Vassilis Angelopoulos awarded NASA Outstanding Public Leadership Medal
Members of the ESP group gathered to celebrate
lease join us in congratulating Professor Vassilis Angelopoulos on receiving the NASA Outstanding Public Leadership Medal for 2024. The Agency Honor Awards are NASA’s most prestigious form of recognition and are presented to individuals and teams who have distinguished themselves by making outstanding contributions to the Agency’s mission. Professor Angelopoulos is receiving the Public Leadership Medal in recognition of his outstanding leadership generating cutting edge Heliophysics research and educating, cultivating, and inspiring a new generation of heliophysicists.
Vassilis Angelopoulos has been a professor in the UCLA Earth, Planetary and Space Sciences Department and Institute of Geophysics and Planetary Physics since 2007. His primary areas of scientific research are how particles are accelerated in Earth's magnetosphere, how the upper atmosphere and ionosphere respond to space currents, and how the lunar environment is affected by its interaction with the solar wind. As Principal Investigator of NASA’s THEMIS and ARTEMIS missions, he led the development of the associated five satellites and twenty ground-based observatories. At UCLA he proposed, oversaw the development, successful launch, and operation of the two ELFIN CubeSats until their deorbit in September 2022. Since its inception, >400 undergraduates have been trained on ELFIN under his and his group’s mentorship.
February 2, 2024:
THEMIS researchers use citizen science video to study new features:
Citizen scientist photo of STEVE, providing researchers with unprecedented detail of its fine structure. The image was selected as the cover of the Journal of Geophysics Research. Credit: Alan Dyer.
A recent article published in Science News details the captivating yet mysterious phenomenon known as STEVE (Strong Thermal Emission Velocity Enhancement), a rare sky glow observed by citizen scientists like Alan Dyer in rural Alberta, Canada. Unlike traditional auroras, STEVE appears as a ribbon of mauve closer to the equator and exhibits unique characteristics. Dyer's high-resolution footage of STEVE provided scientists, particularly THEMIS researcher Toshi Nishimura from Boston University, with unprecedented details. The video revealed a flickering torrent of purplish-white fuzz that challenged existing understandings of atmospheric chemistry.
Scientists have been grappling with the enigmatic nature of STEVE since its introduction to the scientific community in 2016. While initially thought to be related to auroras, STEVE's distinct purple hue and different behavior have raised numerous questions about its origins and underlying mechanisms. Recent observations suggest a connection between STEVE and another atmospheric phenomenon called stable auroral red (SAR) arcs, indicating a complex interplay of factors contributing to STEVE's appearance.
Further complicating the understanding of STEVE is the discovery of its association with green stripes known as the "picket fence," which was initially mistaken for a type of aurora. However, detailed analysis suggests that the picket fence's green glow may stem from different processes than traditional auroras, possibly involving atmospheric electric fields. Computer modeling and proposed rocket missions aim to shed light on these phenomena and confirm the existence of such electric fields.
NASA's upcoming missions, including the Geospace Dynamics Constellation mission, hold promise for gathering more data to elucidate the mysteries surrounding STEVE and related atmospheric phenomena. Despite the challenges, citizen scientists like Dyer remain instrumental in capturing observations of STEVE from the ground, contributing valuable data that continue to puzzle and intrigue researchers in the field of space physics.
Source:
Temming, M. (2023), STEVE and other aurora-like glows perplex scientists with their complex physics, Science News, Published December 2023.
Citation:Nishimura, Y., Dyer, A., Donovan, E. F., Angelopoulos, V. (2023). Fine-scale structures of STEVE revealed by 4K imaging. Journal of Geophysical Research: Space Physics, 128, e2023JA032008. https://doi.org/10.1029/2023JA032008
September 3, 2023:
THEMIS observations of magnetopause Rayleigh-Taylor instability selected as JGR cover:
Artist rendition of the Rayleigh-Taylor Instability at Earth's Magnetopause, when the solar wind dynamic pressure suddenly drops. Credit: G.Q. Yan
The magnetopause, the outermost edge that our planet's intrinsic magnetic field reaches, is always in motion. The macroscopic acceleration at the magnetopause is sometimes as large as a few km/s2. Theoretically, such an acceleration of the boundary can facilitate an instability in the motion of the interface, the Rayleigh-Taylor (R-T) instability. Such an instability can, in turn, cause a transfer of plasma across the magnetopause, from solar wind into near-Earth space. If the resulting particles are sufficiently energetic, operations of many satellites in space can be threatened. However, details of how the instability operates at the magnetopause have been neither verified nor observed in spacecraft observations. Recent measurements from NASA's THEMIS mission indicate that the R-T instability can be excited at the magnetopause, and many details of the instability, including those of its electric field and plasma transport across the magnetopause, appear to be verified in those observations.
For more detail please read the THEMIS nugget summary here or the paper linked below.
Citation:
Yan, G. Q., Parks, G. K., Mozer, F. S., Goldstein, M. L., Chen, T., & Liu, Y. (2023). Rayleigh-Taylor instability observed at the dayside magnetopause under northward interplanetary magnetic field. Journal of Geophysical Research: Space Physics, 128, e2023JA031461. https://doi.org/10.1029/2023JA031461
May 15, 2023:
Kelvin-Helmholtz waves shown to influence equinox geomagnetic activity:
Congratulations to Shiva Kavosi et al., for their recent paper on KH-waves and their influence on equinoctial geomagnetic activity, selected as an Editor's Highlight in Nature Communications. Using THEMIS observations across more than a solar cycle was key to the discovery.
More accurate space-weather predictions and safer satellite navigation through radiation belts could someday result from new insights into “space waves,” researchers at Embry-Riddle Aeronautical University reported. The group’s latest research, published on May 4, 2023, by the journal Nature Communications, shows that seasonal and daily variations in the Earth’s magnetic tilt, toward or away from the Sun, can trigger changes in large-wavelength space waves.
The simulation (right) shows the Earth’s magnetic environment during the equinox and the solstice. As the solar wind – a flow of particles from the Sun – hits the Earth’s magnetic environment, it can create breaking waves known as Kelvin-Helmholtz waves. This occurs more often during the equinoxes due to the orientation of the Sun’s and Earth’s magnetic fields (left). Credits: Shiva Kavosi, ERAU
These breaking waves, known as Kelvin-Helmholtz waves, occur at the boundary between the solar wind and the Earth’s magnetic shield. The waves happen much more frequently around the spring and fall seasons, researchers reported, while wave activity is poor around summer and winter. As plasma or solar wind streams from the Sun at speeds up to 1 million miles per hour, it pushes energy, mass and momentum toward the planet’s magnetic shield. It also whips up space waves. Fast-moving solar wind can’t pass directly through the Earth’s magnetic shield, so it thunders along the magnetosphere, propelling Kelvin-Helmholtz waves with massive peaks up to 15,000 kilometers (km) high and 40,000 km long.
Astronaut Safety and Satellite Communication
“Through these waves, solar wind plasma particles can propagate into the magnetosphere, leading to variations in radiation belt fluxes of energetic particles—regions of dangerous radiation—that may affect astronaut safety and satellite communications,” said Dr. Shiva Kavosi, a research associate at Embry-Riddle and first author of the “Nature Communications” paper. “On the ground, these events can impact power grids and Global Positioning Systems.” Describing the properties of space waves and the mechanisms that cause them to intensify is key to understanding and forecasting space weather, Kavosi noted: “Space weather events represent an increasing threat, yet in many cases, we don’t understand exactly what controls it. Any progress we can make in understanding the mechanisms behind space weather disturbances will improve our ability to provide forecasts and warnings.”
In trying to understand the causes of seasonal and diurnal variations of geomagnetic activity, researchers in the field have set forth several different hypotheses. For example, the Russell-McPherron (R-M) effect, first described in 1973, explains why auroras are more frequent and brighter in the spring and fall, based on the interplay of the Earth’s dipole tilt and a small magnetic field near the Sun’s equator. “We don’t have all the answers yet,” said Dr. Katariina Nykyri, professor of physics and associate director for the Center of Space and Atmospheric Research at Embry-Riddle, “but our paper shows that the R-M effect is not the only explanation for the seasonal variation of geomagnetic activities. Equinox-driven events, based on the Earth’s dipole tilt, and R-M effects could operate simultaneously.”
In the future, Nykyri added, constellations of spacecraft in the solar wind and magnetosphere could more fully explain the complicated, multi-scale physics of space weather phenomena. “Such a system would allow advanced warnings of space weather to inform the operators of rocket launches and electrical power grids,” she said. The “Nature Communications” paper concludes that “KH waves activity exhibit seasonal and diurnal variations, indicating the critical role of dipole tilt in modulating KHI across the magnetopause as a function of time.”
Source:
Anon. (2023), `Space waves' offer new clues to space weather, Embry Riddle Newsroom, Published on May 4, 2023.
Johnson-Groh, M. (2023), NASA Spacecraft Reveal How Earth’s Tilt Causes Seasons in Space Weather, NASA Sun Spot, Published on May 15, 2023.
Citation:
Kavosi, S., Raeder, J., Johnson, J.R., Nykyri, K., Farrugia, C.J. (2023) Seasonal and diurnal variations of Kelvin-Helmholtz Instability at terrestrial magnetopause. Nat Commun 14, 2513. https://doi.org/10.1038/s41467-023-37485-x
April 17, 2023:
Listen to THEMIS data using HARP Citizen Science web app:
Congratulations to Mike Hartinger et al., for the launch of their new NASA Citizen Science web app called HARP, highlighted in Washington Post and NPR.
An international team of researchers invites the public to help in the search for space weather signals: magnetic vibrations around Earth called plasma waves. This new project, Heliophysics Audified: Resonances in Plasmas, or HARP, has just released a web app that takes THEMIS satellite magnetic data and converts it into sounds that the public can listen to and classify.
Learn about the HARP project, where you can help researchers identify plasma waves and improve their models of space weather. Credit: HARP Team, NASA, NCSA-AVL-UIUC, U. Calgary
Plasma waves arise when charged particles from the sun impact Earth's magnetic field, causing it to vibrate or resonate, unleashing radiation that can potentially damage spacecraft. On the brighter side, plasma waves can also generate the beautiful northern and southern lights. Results from the HARP analysis can be fed into space weather models to improve predictions and help clarify the mysterious processes that govern the auroras.
Plasma waves in Earth's environment are invisible, but they can influence the formation of the aurora. Pulsating and shimmering regions appear when charged particles impact Earth's upper atmosphere, making it glow. Credit: Auroral Imaging Group, U. Calgary
"Earth's magnetic field and plasma waves are invisible to cameras," said Mike Hartinger, principal investigator at SSI and a researcher in the UCLA Department of Earth, Planetary, and Space Sciences (EPSS). "HARP uses data collected by NASA's THEMIS satellites traveling through the changing magnetic environment around our planet, where plasma waves can form."
Just as seismologists use earthquake vibrations to "see" inside the Earth, HARP uses magnetic vibrations to investigate how space weather evolves around our planet in response to the Sun's activity.
Plasmas are charged particles that emanate from the sun, which blows a solar wind in continuous streams and explosive eruptions. When the solar wind impacts Earth's magnetic field, different types of plasma waves can form, analogous to vibrations formed by different musical instruments. Credit: Q.-G. Zong, Peking Univ.; M. O. Archer, Imperial College London; M. Hartinger, SSI, UCLA EPSS
HARP expands upon a pilot project in the UK led by team member Martin Archer of Imperial College London, in which high school students found a new complex pattern of plasma waves and became co-authors in a peer-reviewed journal publication. Studies show that people's eyes and ears in combination are better at finding hidden wave patterns than using computer algorithms or eyes alone. Anyone can work with NASA satellite data with just a few minutes of training and make an important contribution to space science.
"We need the public's help to identify plasma waves in huge datasets gathered over the past 16 years by NASA's THEMIS satellites," said Hartinger.
HARP users will be greeted with a brief tutorial, where they listen to the satellite data simultaneously while viewing it in a graph of frequency and intensity vs. time, called a spectrogram. Users can draw boxes around interesting features and describe their characteristics, such as changes in pitch or whether there are single or multiple tones, and jot down any other observations.
Although HARP currently uses only one satellite mission, the next step is to add other heliophysics satellites and data sources to incorporate more types of plasma waves throughout the solar system. The public holds the key to the success of HARP and can hasten future discoveries, visit listen.spacescience.org and sign up today!
Source:
Archer, M.O., Nykyri, K. (2023), Listen to the Sounds of Space, ISSI Bern, Published on April 17, 2023.
Baker, H. (2023), Eerie sounds triggered by plasma waves hitting Earth's magnetic field captured in new NASA sound clip, Livescience, Published on April 18, 2023.
Sources:
Blakemore, E., (2023), Want to listen to space noise? NASA wants to hear from you, Washington Post, Published on Apr. 23, 2023.
Hoeflinger, J. (2023), Imperial researchers team up with NASA to launch new citizen science study, Imperial College London, Published on April 17, 2023.
Kwong, E., Barber, R.G. (2023), From 'Short Wave': magnetosphere music, Jupiter's icy moons and a runaway black hole, aired on NPR All Things Considered on April 20, 2023.
Masongsong, E. (2023), UCLA Scientists Inviting People to Explore Space With Their Ears, UCLA EPSS website, Published on April 19, 2023.
Matthew, Z. (2023), NASA and UCLA want you to help identify the sounds of space, KCRW Greater LA Podcast, Published on May 4, 2023.
Thomas, V. (2023), Help Discover the Sounds of Space Played by NASA’s HARP, NASA website, Published on April 17, 2023.
April 1, 2023:
THEMIS researcher talks about STEVE in Washington Post:
Congratulations to Toshi Nishimura et al. for receiving quotes and a reference to their Frontiers paper using THEMIS data, featured in a recent article on STEVE in the Washington Post.
STEVE is not an aurora, but you can think of it as a shy, distant cousin. It looks as if it could be part of the family, but it has its own distinct style. The phenomenon generally appears as a long, slender purple-and-white arc, sometimes accompanied by a structure that looks like a green picket fence. It is fainter and narrower, and occurs at lower latitudes than most auroras. It is also harder to predict.
What are some impacts of STEVE? Even if you haven’t seen the awe-inspiring STEVE, its impacts could be experienced by Earthlings in other ways.
STEVE researcher Toshi Nishimura recounted at least one instance in which a radio signal from a radar network disappeared for 30 minutes as STEVE appeared, but then returned once the ghostly light left the area, suggesting that the phenomenon can disrupt such signals. Similar blackouts can occur with space weather associated with auroras, but he said STEVE occurs in a different region. He said it "would have a larger impact on densely populated regions at lower latitudes than aurora.”
STEVE is important because Earth’s magnetic field “is doing something different from usual. … We still don’t understand why,” said Nishimura, who recently published a study on the mysteries of STEVE. He has yet to see the phenomenon in person.
A photo of STEVE captured in Plumas in August 2022. STEVE is often accompanied by a green picket fence structure, appearing here at the lower edge of the pink arc. (Donna Lach)
Nishimura, a researcher at Boston University, said STEVE seems to always occur during local and brief disturbances in Earth’s magnetic field, called substorms. Substorms can occur without a large geomagnetic storm and happen daily. Yet despite the ubiquity of substorms, researchers are perplexed about why STEVE sightings are still relatively rare compared with auroras.
With every photo and report, people are understanding more about this relatively unexplored part of our atmosphere, and its connection with the sun.
Source:
Patel, K., (2023), How to find STEVE, the purple streak that looks like an aurora but isn’t, Washington Post, Published on Apr. 1, 2023.
Citation:Nishimura, Y. et al. (2023), Unsolved problems in Strong Thermal Emission Velocity Enhancement (STEVE) and the picket fence, Front. Astron. Space Sci., 10, https://doi.org/10.3389/fspas.2023.1087974
February 1, 2023:
THEMIS data reveals lunar tide in the plasmasphere:
Congrats to Xiao et al. for their discovery of a lunar tide effect on the Earth's plasmapause. For their paper published in the journal Nature Physics, the group used data from multiple spacecraft including THEMIS-ARTEMIS and Van Allen Probes over a nearly 40-year period to measure tidal perturbations in the plasmapause.
Early scientists found a connection between the tides and the movement of the moon thousands of years ago. More recent evidence suggests the moon's pull acts on the ionosphere as well. In this new study, the researchers wondered if the moon might also have an impact on the plasmasphere. The plasmasphere is a toroidal mass of plasma that surrounds the Earth. It lies beyond the ionosphere and is made up mostly of electrons and protons. Its particles are charged by the ionosphere, and its outer boundary is known as the plasmapause. To find out if it is impacted by the moon's gravity, the researchers obtained data from approximately 36,000 crossings of the plasmasphere by spacecraft over the years 1977 to 2015. They also used data from the Van Allen Probes from the years 2012 to 2019 to gain a better perspective on possible plasmasphere modulations.
Ocean tides (blue) compared to plasmapause tides (orange). Credit: Q.Q. Shi, Shandong University (video link)
The group found that they were able to isolate tidal variations in the shape of the plasmapause that could be associated with the position of the moon, clear evidence that the moon does exert an influence on the plasmasphere. They also found that they were able to see monthly periodicities in the changes in plasmapause. The researchers propose that three basic elements are responsible for the tidal variations: the existence of a two-body system—namely the Earth and moon—along with the existence of the plasma field and the existence of the magnetic field. They further suggest that similar tidal variations likely occur in other two-body systems throughout the universe.
Source:
Yirka, B., (2023), Evidence found of tidal impact on the plasmasphere, Phys.org, Published on Feb. 1, 2023.
Xiao, C. et al. (2023), Moon can cause a tide in Earth’s “plasma ocean," Nature Portfolio Astronomy Community, Published Jan. 27, 2023.
Phillips, T. (2023), Strange tides in the plasmasphere, spaceweather.com, Published on Feb. 8, 2023.
Hua, X. (2023), Scientists find evidence of lunar tide impact on plasmasphere, China Daily, Published Feb. 7, 2023.
Luntz, S. (2023), Even The Earth’s Magnetic Field Has Moon-Driven Tides, IFL Science, Published Feb. 6, 2023.
Stan, J. (2023), Moon Exerts Obscure Tidal Force 'Plasma Ocean' That Creates Fluctuation on Earth's Magnetosphere, Study Reveals, Science Times, Published Feb. 3, 2023.
Citation:Xiao, C. et al. (2023), Evidence for lunar tide effects in Earth's plasmasphere, Nature Physics. DOI: 10.1038/s41567-022-01882-8
October 7, 2022:
Space Raindrops Splashing on Earth’s Magnetic Umbrella:
Congrats to Vuorinen et al. for their recent feature article in Eos magazine, profiling THEMIS-ARTEMIS measurements of magnetosheath jets and their influence on magnetic reconnection.
Though not as damaging as extreme space weather events, showers of plasma jets hit Earth’s magnetic shield every day—yet we’re only beginning to understand their effects. Every few minutes, Earth-sized “droplets” of plasma rain down from space toward Earth. Instead of crashing catastrophically to the ground, these droplet/s, called magnetosheath jets, hit and are deflected by the outer reaches of Earth’s magnetic field.
Despite their frequent occurrence near Earth and likely ubiquity across the solar system, the study of magnetosheath jets is young, and there is much we do not know about their origins and behavior. Specifically, their potential effects on space weather—the phenomena we experience on Earth due to the ever-changing stream of plasma that flows through our solar system—are unclear and still being investigated. Therefore, these jets are not currently factored into space weather models or predictions. Here we discuss recent findings in this field and important questions that remain to be answered.
Fig. 1. The solar wind with its interplanetary magnetic field (yellow lines) flows from the Sun and forms a shock wave called the bow shock (bright orange line) where it meets Earth’s magnetic field upstream of the planet. At the bow shock, the solar wind is slowed, except for fast plasma jets (orange globules) emerging from where the shock wave is corrugated, adjacent to the turbulent foreshock region (brown shading at top right). Jets travel through the magnetosheath (red swirls), and eventually, some of them impact the magnetopause (bright blue line), the outer edge of Earth’s magnetosphere (blue shading). Earth’s magnetic field (faint blue lines) and an aurora in Earth’s ionosphere are also represented. Credit: E. Masongsong, H. Hietala, L. Vuorinen, A. LaMoury
Our knowledge of magnetosheath jets relies on data captured by spacecraft surveying the near-Earth electromagnetic environment. Launched in 2007 and still going strong, NASA’s THEMIS-ARTEMIS five-satellite mission in particular has greatly enhanced our understanding of many areas of space and space weather, from the solar wind and its interactions with Earth’s magnetic field to the aurora and even plasmas around the Moon.
In a recent study using THEMIS data, researchers observed that the frequency of jets reaching the magnetopause is highly dependent on the properties of the solar wind at the bow shock [LaMoury et al., 2021]. They found that when the solar wind’s magnetic field is nearly radial, jets are expected to hit the magnetopause every few minutes—that is, over 17 times more often than when the solar wind’s magnetic field reaches Earth at an oblique angle. They also found that more jets arose during intervals of “fast” solar wind (which travels about twice the normal velocity). These intervals vary with the 11-year solar cycle, so the number of jets hitting the magnetopause may exhibit the same periodic dependence.
In addition to controlling the structure of different bow shock regions, the orientation of the solar wind magnetic field influences what happens at the magnetopause. Zooming in on this boundary where the solar wind magnetic field meets Earth’s magnetic field, we see that geometry comes into play once again.
Namely, if the solar wind magnetic field is oriented southward, opposite to Earth’s northward magnetic field, erosion of the magnetopause can occur via a process known as magnetic reconnection (Figure 2). Reconnection is a key process in plasma physics, in which the connectedness of magnetic field lines is fundamentally changed and energy stored in the magnetic field is explosively released.
In the case of the magnetopause, antiparallel field lines of the solar wind connect with those of Earth, peeling them away from the Sun-facing side of the magnetosphere. This has the effect of weakening Earth’s magnetic shield, allowing solar wind plasma and energy to enter Earth’s magnetosphere. Thus, one of the most critical aspects of forecasting space weather is monitoring the solar wind magnetic field orientation far upstream of Earth.
Fig. 1. The solar wind with its interplanetary magnetic field (yellow lines) flows from the Sun and forms a shock wave called the bow shock (bright orange line) where it meets Earth’s magnetic field upstream of the planet. At the bow shock, the solar wind is slowed, except for fast plasma jets (orange globules) emerging from where the shock wave is corrugated, adjacent to the turbulent foreshock region (brown shading at top right). Jets travel through the magnetosheath (red swirls), and eventually, some of them impact the magnetopause (bright blue line), the outer edge of Earth’s magnetosphere (blue shading). Earth’s magnetic field (faint blue lines) and an aurora in Earth’s ionosphere are also represented. Credit: E. Masongsong, H. Hietala, L. Vuorinen, A. LaMoury
Although measuring the north–south component of the solar wind magnetic field before it arrives to Earth (together with solar wind speed and density) can help us prepare for space weather storms, it does not give us the full picture of how space weather evolves at Earth. Much more frequent rain showers (i.e., jets) may play a role that, until recently, had not been considered to control magnetic reconnection, sparking debate in the magnetospheric physics community. Jets, because of their high dynamic pressure, have been observed compressing the magnetopause boundary to help initiate reconnection, as opposite magnetic fields are being pushed together more efficiently [Hietala et al., 2018].
In addition, researchers have suggested that the magnetic field orientation in jets may be different from field orientations in the surrounding magnetosheath. Nykyri et al. [2019] observed jets with southward magnetic fields propagating toward the magnetopause and triggering reconnection despite measurements at the time showing the solar wind magnetic field was oriented northward. This was likely not an isolated occurrence, and it caused a chain of events leading to increased geomagnetic activity on the nightside of the magnetosphere, opposite from the Sun.
Vuorinen et al. [2021] performed a statistical study of the north–south component in jets versus the surrounding magnetosheath using THEMIS data, further confirming that jets often carry southward magnetic fields even when the solar wind magnetic field is northward. The combination of jets compressing and introducing southward fields to the magnetopause may be favorable for triggering reconnection, even during solar wind conditions when we are not typically expecting it. This result highlights the importance of studying the magnetosphere’s behavior during times of a radial interplanetary magnetic field.
Source:
Vuorinen, L., A. LaMoury, E. Masongsong, and H. Hietala (2022), Space raindrops splashing on Earth’s magnetic umbrella, Eos, 103, https://doi.org/10.1029/2022EO220485. Published on 7 October 2022..
Citation:Hietala, H., et al. (2018), In situ observations of a magnetosheath high-speed jet triggering magnetopause reconnection, Geophys. Res. Lett., 45, 1,732–1,740, https://doi.org/10.1002/2017GL076525.
LaMoury, A. T., et al. (2021), Solar wind control of magnetosheath jet formation and propagation to the magnetopause, J. Geophys. Res. Space Phys., 126, e2021JA029592, https://doi.org/10.1029/2021JA029592.
Nykyri, K., et al. (2019), Can enhanced flux loading by high-speed jets lead to a substorm? Multipoint detection of the Christmas Day substorm onset at 08:17 UT, 2015, J. Geophys. Res. Space Phys., 124, 4,314–4,340, https://doi.org/10.1029/2018JA026357.
Plaschke, F., et al. (2018), Jets downstream of collisionless shocks, Space Sci. Rev., 214, 81, https://doi.org/10.1007/s11214-018-0516-3.
Raptis, S., et al. (2022), Downstream high-speed plasma jet generation as a direct consequence of shock reformation, Nat. Commun., 13, 598, https://doi.org/10.1038/s41467-022-28110-4.
Vuorinen, L., et al. (2021), Magnetic field in magnetosheath jets: A statistical study of Bz near the magnetopause, J. Geophys. Res. Space Phys., 126, e2021JA029188, https://doi.org/10.1029/2021JA029188.
June 2, 2022:
CLUSTER-THEMIS-MMS observations contribute to big picture of auroral beads :
Congrats to Petrinec et al. for their recent publication profiling magnetopause vortices and auroral bead formation with 13 spacecraft, including THEMIS, CLUSTER, MMS, Geotail and DMSP.
One solar stormy day in November 2018, 13 spacecraft including ESA’s Cluster mission were in the right place at the right time to spot a process that has never been seen in its entirety before. Their observations explain how vortices at the edge of Earth’s magnetosphere can cause auroral beads to dot the sky a hundred thousand kilometres below.
This connection between auroral beads appearing on Earth’s ‘dayside’ (or Sun-facing side) and the vortices confirms a theory about how these unique auroras – known as beads because they look like a string of pearls hung across the sky – form. As some spacecraft observed the vortices themselves, others saw that a stream of charged particles used the vortices as access points to tunnel down towards Earth’s surface, causing the sky to glow.
Using multi-spacecraft observations, researchers explain how vortices at the edge of Earth’s magnetosphere can cause auroral beads to dot the sky a hundred thousand kilometres below: 1) The solar wind 'blows' across the magnetopause, rolling it up into giant whirlpool-like vortices. 2) Electrons enter the magnetosphere through the vortices and travel down towards the upper atmosphere. 3) In the upper atmosphere, electrons collide with hydrogen, oxygen and nitrogen, causing auroral beads to glow in the sky.
On 6 November 2018, 12 spacecraft were all located close to the magnetopause – the thin boundary at the outer edge of the magnetosphere – on the night side of Earth, where the magnetosphere stretches out into a tail.
Among the 12 spacecraft located close to the magnetosphere were the four that make up ESA’s Cluster mission, as well as NASA’s four Magnetospheric Multiscale (MMS) spacecraft and three Time History of Events and Macroscale Interactions during Substorms (THEMIS) spacecraft, and JAXA’s Geotail. In addition, a US Defence Meteorological Satellite Program (DMSP) satellite observed the auroral beads from close to Earth’s surface.
“This discovery shows that the Cluster spacecraft are part of a ‘magnetospheric orchestra’ of missions that together enable extra science that is not possible to achieve with each mission individually,” explains Philippe Escoubet, ESA’s Cluster project scientist.
The vortices – which themselves were originally detected by Cluster – are formed when the solar wind blows past the magnetopause. Just as wind on Earth can whip up oceans and clouds, the solar wind can roll up the magnetopause into giant waves comprised of whirlpool-like vortices.
When a vortex is at the ‘goldilocks’ size – not too big, but not too small – electrons from the solar wind swirl around its centre, before entering the magnetosphere, travelling towards and reaching Earth’s upper atmosphere. There, the electrons collide with hydrogen, oxygen and nitrogen, causing them to glow and form an auroral bead in the sky. These round beads – one for each vortex – appear in groups that follow each other across the sky. This is in contrast to ‘normal’ aurora which are flatter, more elongated and not so well organised.
Philippe continues: “Cluster has been operating for almost 22 years now. In the beginning, it was one of the only missions observing the magnetosphere, so we were mainly comparing the four spacecraft with each other. But nowadays we can compare their data with those from other missions, such as MMS and THEMIS.”
This research demonstrates the importance of multiple different spacecraft, each with their own complement of scientific instruments, monitoring the same events from different vantage points.
Source:
ESA, Magnetic vortices explain mysterious auroral beads, June 2, 2022.
Space.com
Citation:Petrinec, S. et al. (2022), Multi-Spacecraft Observations of Fluctuations Occurring Along the Dusk Flank Magnetopause, and Testing the Connection to an Observed Ionospheric Bead, Frontiers in Astronomy and Space Sciences. DOI: 10.3389/fspas.2022.827612
March 29, 2022:
Winner of GEM Outstanding Student Poster Award:
EPSS Space Physics graduate student Colin Wilkins won an award for best Inner Magnetosphere Section Poster at the 2022 Geospace Environment Modeling (GEM) Summer Workshop last week. Colin's posted was titled "Statistical Characterization of the Electron Isotropy Boundary from ELFIN Observations."
The GEM meeting is the premiere topical conference in Magnetospheric Physics; this year it attracted about 320 people, including more than 100 of them students from around the world. Colin’s was one of a handful selections, in the most competitive section. Congratulations!
March 29, 2022:
THEMIS-ELFIN observations of whistler wave electron precipitation :
UCLA scientists have discovered a new source of super-fast, energetic electrons raining down on Earth’s atmosphere, a phenomenon that contributes to the colorful aurora borealis but also poses hazards to satellites, spacecraft and astronauts.
The researchers observed unexpected, rapid “electron precipitation” from low-Earth orbit using the ELFIN mission, a pair of tiny satellites built and operated on the UCLA campus by undergraduate and graduate students guided by a small team of staff mentors.
By combining the ELFIN data with more distant observations from NASA’s THEMIS spacecraft, the scientists determined that the sudden downpour was caused by whistler waves, a type of electromagnetic wave that ripples through plasma in space and affects electrons in the Earth’s magnetosphere, causing them to “spill over” into the atmosphere.
The THEMIS and ELFIN satellites (orbits shown in cyan and green, respectively) worked together to help understand the mystery of electron rain. When whistler waves (purple) interact with the electrons, they can give them extra energy (red spiral), which causes them to fall into the atmosphere.
Their findings, published March 25 in the journal Nature Communications, demonstrate that whistler waves are responsible for far more electron rain than current theories and space weather models predict.
“ELFIN is the first satellite to measure these super-fast electrons,” said Xiaojia Zhang, lead author and a researcher in UCLA’s department of Earth, planetary and space sciences. “The mission is yielding new insights due to its unique vantage point in the chain of events that produces them.”
Central to that chain of events is the near-Earth space environment, which is filled with charged particles orbiting in giant rings around the planet, called Van Allen radiation belts. Electrons in these belts travel in Slinky-like spirals that literally bounce between the Earth’s north and south poles. Under certain conditions, whistler waves are generated within the radiation belts, energizing and speeding up the electrons. This effectively stretches out the electrons’ travel path so much that they fall out of the belts and precipitate into the atmosphere, creating the electron rain.
One can imagine the Van Allen belts as a large reservoir filled with water — or, in this case, electrons. As the reservoir fills, water periodically spirals down into a relief drain to keep the basin from overflowing. But when large waves occur in the reservoir, the sloshing water spills over the edge, faster and in greater volume than the relief drainage. ELFIN, which is downstream of both flows, is able to properly measure the contributions from each flow.
The low-altitude electron rain measurements by ELFIN, combined with the THEMIS observations of whistler waves in space and sophisticated computer modeling, allowed the team to understand in detail the process by which the waves cause rapid torrents of electrons to flow into the atmosphere.
The findings are particularly important because current theories and space weather models, while accounting for other sources of electrons entering the atmosphere, do not predict this extra whistler wave–induced electron flow, which can affect Earth’s atmospheric chemistry, pose risks to spacecraft and damage low-orbiting satellites.
The researchers further showed that this type of radiation belt electron loss to the atmosphere can increase significantly during geomagnetic storms, disturbances caused by enhanced solar activity that can affect near-Earth space and Earth’s magnetic environment.
Although space is commonly thought to be separate from our upper atmosphere, the two are inextricably linked. Understanding how they’re linked can benefit satellites and astronauts passing through the region, which are increasingly important for commerce, telecommunications and space tourism.
Since its inception in 2013, more than 300 UCLA students have worked on ELFIN (Electron Losses and Fields investigation), which is funded by NASA and the National Science Foundation. The two microsatellites, each about the size of a loaf of bread and weighing roughly 8 pounds, were launched into orbit in 2018, and since then have been observing the activity of energetic electrons and helping scientists to better understand the effect of magnetic storms in near-Earth space. The satellites are operated from the UCLA Mission Operations Center on campus.
“It’s so rewarding to have increased our knowledge of space science using data from the hardware we built ourselves,” said Colin Wilkins, a co-author of the current research who is the instrument lead on ELFIN and a space physics doctoral student in the department of Earth, planetary and space sciences.
Source: Masongsong, E. (2022), UCLA researchers discover source of super-fast ‘electron rain.' UCLA Newsroom, March 29, 2022.
India Education Diary
Innovations Report
Notimerica/Europe Press
SciTech Daily
Nature Comm. Editor's Highlight
Nature Comm. Behind the Paper Blog Post
Citation: Zhang, X.-J., Artemyev, A. V., Angelopoulos, V., Tsai, E., Wilkins, C., Kasahara, S., Mourenas, D., Yokota, S., Keika, K., Hori, T., Miyoshi, Y., Shinohara, I., Matsuoka, A. (2022), Superfast precipitation of energetic electrons in the radiation belts of the Earth, Nat. Comm., 13, 1611, https://doi.org/10.1038/s41467-022-29291-8
March 11, 2022:
THEMIS-ASI Auroral Beads selected as JGR cover:
Congrats to Nishimura et al. for their recent JGR paper being chosen for the cover. The research utilized simultaneous THEMIS ASI and satellite observations to explain how plasma flows create auroral beads, in the context of substorm auroral intensification.
Aurora in the night sky often begins with a sudden intensification called a substorm, and the auroral intensification shows a wave-like display (beads). It is critical to understand the origin of beads for revealing the mechanism of the sudden auroral intensifications, but it has been extremely difficult to find satellite observations in space to explore the magnetospheric source region of substorms.
Image shows an aurora which was observed by an all-sky camera at Fort Yukon, Alaska, at 9:14 UT on 20 November 2014. The image is projected onto the geographic map in Alaska. North is to the top and east is to the right. The image shows wave-like auroral structures called auroral beads. Credit: NASA/CSA/University of California, Berkeley/University of Calgary/NSF.
Nishimura et al. identified two simultaneous observations of auroral beads at the beginning of substorm auroral intensification, using all-sky imagers and related plasma dynamics by the THEMIS satellites in the plasma sheet. They found that the bead structure and propagation are related to charge accumulation and fast plasma streams in the plasma sheet. The plasma flow speed coincided with the bead speed, and the charge distribution and plasma stream direction reversed when the auroral beads moved in the opposite direction, giving strong evidence of the connection between the observations at the two locations. The plasma streams reduced the electric currents in the plasma sheet, and the paper suggests that the current reduction destabilizes the plasma sheet and initiates the substorm auroral intensification.
October 6, 2021:
Researchers Find Standing Waves at Edge of Earth's Magnetic Bubble:
Congrats to Martin Archer et al. for their recent publication in Nature Communications. Using a combination of theory, THEMIS observations and global simulations, they discovered magnetopause standing waves propagating against the flow of the solar wind.
Earth sails the solar system in a ship of its own making: the magnetosphere, the magnetic field that envelops and protects our planet. The celestial sea we find ourselves in is filled with charged particles flowing from the Sun, known as the solar wind. Just as ocean waves follow the wind, scientists expected that waves traveling along the magnetosphere should ripple in the direction of the solar wind. But a new study reveals some waves do just the opposite.
An animated illustration of magnetospheric waves, in light blue. At the front of the magnetosphere, these waves appear to be still. Credits: M. Archer/E. Masongsong/NASA
Studying these magnetospheric waves, which transport energy, helps scientists understand the complicated ways that solar activity plays out in the space around Earth. Changing conditions in space driven by the Sun are known as space weather. That weather can impact our technology from communications satellites in orbit to power lines on the ground. “Understanding the boundaries of any system is a key problem,” said Martin Archer, a space physicist at Imperial College London who led the new study, published today in Nature Communications. “That’s how stuff gets in: energy, momentum, matter.”
Archer focuses on surface waves, meaning waves that require a boundary — in this case, the edge of the magnetosphere — to travel along. Previously, he and his colleagues established this boundary vibrates like a drum. When a strong burst of solar wind beats against the magnetosphere, waves race towards Earth’s magnetic poles and get reflected back.
The latest work considers the waves that form across the entire surface of the magnetosphere, using a combination of models and observations from NASA’s THEMIS mission, Time History of Events and Macroscale Interactions during Substorms.
The researchers found when solar wind pulses strike, the waves that form not only race back and forth between Earth’s magnetic poles and the front of the magnetosphere, but also travel against the solar wind. Archer likened these two kinds of movement to crossing a river: A boat can go from one riverbank to the other (traveling towards the poles) and upstream (against the solar wind). At the front of the magnetosphere, these waves appear to stand still.
The THEMIS satellites’ observations from within the magnetosphere first hinted some waves might be traveling against the solar wind. The researchers used models to illustrate how the energy of the wind coming from the Sun and that of the waves going against it could cancel each other out. It’s similar to what happens if you try walking up a downwards escalator. “It’s going to look like you’re not moving at all, even though you’re putting in loads of effort,” Archer said.
These standing waves can persist longer than those that travel with the solar wind. That means they’re around longer to accelerate particles in near-Earth space, leading to potential impacts in the radiation belts, aurora, or ionosphere. Archer expects standing waves may occur elsewhere in the universe, from the magnetospheres of other planets to the peripheries of black holes. Studying the waves close to home can help scientists understand such distant boundaries.
By translating the wave models and data into the audible range, we can listen to the sound of these curious waves.
Source:
Tran, L., With NASA Data, Researchers Find Standing Waves at Edge of Earth’s Magnetic Bubble, NASA News, Oct. 6, 2021.
Dunning, H., When the solar wind hits Earth’s magnetosphere, a surprising stillness ensues, Imperial College London, Oct. 6, 2021.
Science Times
SciTech Daily
UK News 18
Citation:
Archer, M. O., Hartinger, M. D., Plaschke, F., Southwood, D. J., Rastaetter, L. (2021), Magnetopause ripples going against the flow form azimuthally stationary surface waves, Nat. Comm., 12, 5697, https://doi.org/10.1038/s41467-021-25923-7
September 24, 2021:
Earth can make auroras without solar activity:
Congrats to Xu Zhang et al. for their feature on Spaceweather.com! Using THEMIS observations and statistical analyses, they revealed an atmospheric electron beam source for ECH waves, which in turn can power the diffuse aurora.
Diffuse auroras and the Big Dipper, photographed by Emmanuel V. Masongsong in Fairbanks, AK
No solar storms? No problem. Earth has learned to make its own auroras. New results from NASA’s THEMIS-ARTEMIS spacecraft show that a type of Northern Lights called "diffuse auroras" comes from our own planet–no solar storms required.
Diffuse auroras look a bit like pea soup. They spread across the sky in a dim green haze, sometimes rippling as if stirred by a spoon. They’re not as flamboyant as auroras caused by solar storms. Nevertheless, they are important because they represent a whopping 75% of the energy input into Earth’s upper atmosphere at night. Researchers have been struggling to understand them for decades.
“We believe we have found the source of these auroras,” says UCLA space physicist Xu Zhang, lead author of papers reporting the results in the Journal of Geophysical Research: Space Physics and Physics of Plasmas.
It is Earth itself.
Earth performs this trick using electron beams. High above our planet’s poles, beams of negatively-charged particles shoot upward into space, accelerated by electric fields in Earth’s magnetosphere. Sounding rockets and satellites discovered the beams decades ago. It turns out, they can power the diffuse auroras.
The video, below, shows how it works. The beams travel in great arcs through the space near Earth. As they go, they excite ripples in the magnetosphere called Electron Cyclotron Harmonic (ECH) waves. Turn up the volume and listen to the waves recorded by THEMIS-ARTEMIS:
ECH waves, in turn, knock other electrons out of their orbits, forcing them to fall back down onto the atmosphere. This rain of secondary electrons powers the diffuse auroras.
"This is exciting," says UCLA professor Vassilis Angelopoulos, a co-author of the papers and lead of the THEMIS-ARTEMIS mission. "We have found a totally new way that particle energy can be transferred from Earth’s own atmosphere out to the magnetosphere and back again, creating a giant feedback loop in space."
According to Angelopoulos, Earth’s polar electron beams1 sometimes weaken but they never completely go away, not even during periods of low solar activity. This means Earth can make auroras without solar storms.
The sun is currently experiencing periods of quiet as young Solar Cycle 25 sputters to life. Pea soup, anyone?
Source: Phillips, T., Earth Can Make Auroras Without Solar Activity, Spaceweather.com, Sept. 30, 2021.
AstroBob Duluth News Tribune
Times UK
Tiempo Meteored Madrid, Spain
Citation:
Zhang, X., Angelopoulos, V., Artemyev, A. V., Zhang, X.-J. (2021), Beam-driven ECH waves: A parametric study, Phys. Plasmas, 28, 072902, https://doi.org/10.1063/5.0053187
Zhang, X., Angelopoulos, V., Artemyev, A. V., Zhang, X.‐J., Liu, J. (2021). Beam‐driven electron cyclotron harmonic waves in Earth’s magnetotail. Journal of Geophysical Research: Space Physics, 126, e2020JA028743. https://doi.org/10.1029/2020JA028743
May 11, 2020:
SpaceNews.com interviews ELFIN about remotely operating CubeSats during Covid-19 Lockdown:
Students operating the twin Electron Losses and Fields Investigation (ELFIN) cubesats were heading into finals at the University of California, Los Angeles, when they realized they needed to quickly transition to remote operations. "As soon as all of us were done taking three-hour tests, we had three-hour meetings to figure out what we needed the satellites to do and how to make it as easy as possible from a software and technical perspective," said Sharvani Jha, ELFIN software development lead.
UCLA students began establishing remote access soon after ELFIN launched in 2018 alongside NASA’s Ice, Cloud and land Elevation Satellite-2. "We have winter break and summer break where we would not be in Los Angeles physically," said Rebecca Yap, ELFIN mission operations manager. "Being able to set up a flexible operations workflow was important."
Link to full article